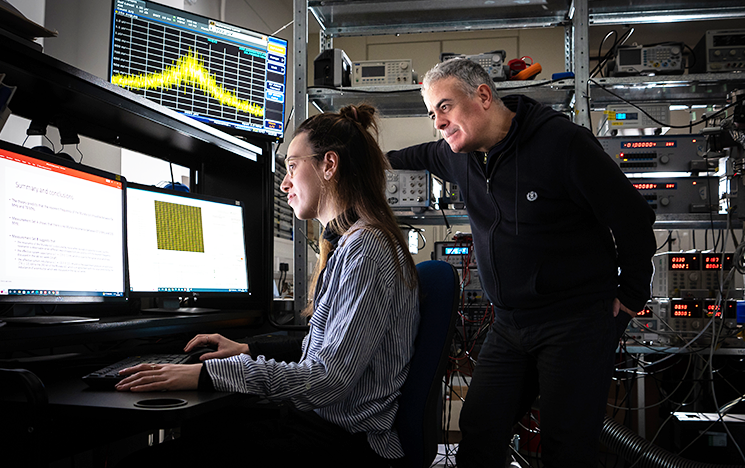
Sussex Centre for Quantum Technologies
Our eight research groups are harnessing and exploiting quantum physics research in order to create transformational commercial products with the potential to change the way we live and work.
Let us inspire you
We work with leading industry partners in commercialising our ground-breaking research. Quantum technologies will provide disruptive capabilities for numerous applications across a range of sectors.
Our specialised facilities and expertise are unique and have given rise to many world firsts. Our Doctoral and Industry Training Academy provides authentic quantum technology training, with an emphasis on entrepreneurship and engineering excellence.
Work with us to carry out research with impact and make the world a better place.
Centre of Excellence
The Sussex Centre for Quantum Technologies is a Centre of Excellence. Our Centres of Excellence are drawing together world-leading experts and innovative approaches, creating a critical mass of knowledge, skills and training – and proving that a challenge is only impossible until it's done.
Who we are
Learn about our research and ways to work with us.
Hear about how we make use of the strange phenomena of quantum physics to build new machines that will change the way we live.
Latest news
Contact us
Email:
SCQT@sussex.ac.uk
Telephone:
+44 (0)1273 872887